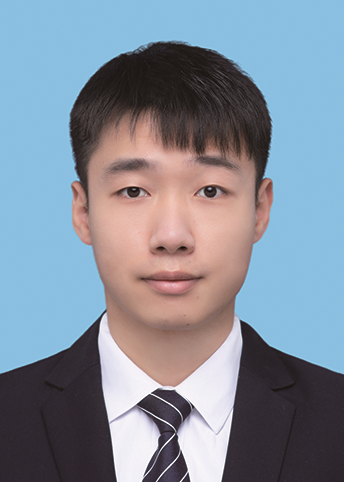
Citation: | Sun LX,Wu S,Zhang XW,et al.Investigation on the growth factor regulatory network of dermal fibroblasts in mouse full-thickness skin defect wounds based on single-cell RNA sequencing[J].Chin J Burns Wounds,2022,38(7):629-639.DOI: 10.3760/cma.j.cn501225-20220215-00029. |
(1)证明硫氧还蛋白还原酶1(TXNRD1)在脓毒症小鼠树突状细胞中表达降低,从而加重细胞铁死亡,这为脓毒症免疫治疗提供了新靶点。
(2)在蛋白水平证实,抑制TXNRD1蛋白表达可加重脓毒症小鼠树突状细胞的脂质过氧化,并影响树突状细胞免疫功能,这为明确脓毒症时细胞免疫功能障碍的关键信号机制提供了新思路。
Highlights:
(1)It was demonstrated that expression of thioredoxin reductase 1 (TXNRD1) in dendritic cells (DCs) of septic mice was reduced, thereby aggravating cell ferroptosis, which provided a new target for sepsis immunotherapy.
(2)It was confirmed at the protein level that inhibiting TXNRD1 protein expression could aggravate lipid peroxidation in septic mouse DCs and affect the immune function of DCs, offering new insights for clarifying the key signaling mechanisms of cellular immune dysfunction in sepsis.
脓毒症是由感染诱发机体反应失调所致严重器官功能障碍,表现为早期的炎症过度活化及晚期持续性免疫抑制,这种免疫失衡和由此进一步引发的MOF是导致脓毒症致死率高的重要原因[1, 2, 3]。树突状细胞(dendritic cell,DC)在免疫系统的启动与调节过程中扮演着核心角色,通过其抗原提呈功能和与T细胞的相互作用调控获得性免疫反应[4, 5, 6, 7]。脓毒症状态下DC呈现明显功能损害,表现为细胞成熟受抑、亚群比例改变,造成免疫应答失调[8, 9, 10]。
铁死亡是一种由铁离子介导的调节性死亡,参与包括肿瘤、神经退行性疾病与脓毒症在内多种病理状态的进展,主要特征为胞内铁超载、谷胱甘肽耗竭及脂质过氧化物的积累[11, 12]。新近研究提示,DC铁死亡是脓毒症发生发展过程中引起免疫功能紊乱的关键因素之一[13, 14]。硫氧还蛋白(thioredoxin,TRX)系统是除谷胱甘肽系统外哺乳动物细胞的另一主要抗氧化机制,依靠二硫键的氧化/还原形态转化以维持细胞氧化还原平衡[15, 16]。TRX还原酶1(thioredoxin reductase 1,TXNRD1)是TRX系统的中枢调节因子,通过协同还原型烟酰胺腺嘌呤二核苷酸磷酸维持TRX的还原功能,在调控细胞应激反应、氧化还原稳态及防止氧化损伤方面发挥关键作用。TXNRD1的功能不仅限于抗氧化效应,还涉及免疫调节、细胞信号传递及细胞死亡调控,其在炎症性疾病免疫细胞的抗氧化反应中可能发挥作用[17, 18, 19]。本研究旨在通过构建脓毒症小鼠模型,探讨脓毒症状态下DC中TXNRD1表达与铁死亡及DC免疫功能的相关性,并进一步探究TXNRD1调控脓毒症状态下DC铁死亡、免疫反应的作用及对脓毒症动物预后的影响,为改善创面感染等引起的脓毒症免疫抑制提供依据。
本实验研究进行动物实验前已通过解放军总医院医学伦理委员会审批,实验过程符合单位及国家的实验动物管理和使用规定。
160只6~8周龄体重20~22 g雄性健康无特殊病原体级C57BL/6J小鼠购自北京华阜康生物科技股份有限公司,许可证号:SCXK(京)2019-0008。
兔抗小鼠TXNRD1多克隆抗体购自深圳优品生物科技有限公司,兔抗小鼠溶质载体家族7成员11(solute carrier family 7 member 11,SLC7A11)单克隆抗体、兔抗小鼠谷胱甘肽过氧化物酶4(glutathione peroxidase 4,GPX4)单克隆抗体购自美国Abcam公司,异硫氰酸荧光素标记的小鼠源性I-A/I-E(主要组织相容性复合体Ⅱ类亚型)抗体、藻红蛋白标记的小鼠源性CD80(白细胞分化抗原)抗体、藻红蛋白/花青素7标记的小鼠源性CD86(白细胞分化抗原)抗体购自美国BioLegend公司,辣根过氧化物酶(horseradish peroxidase,HRP)标记的山羊抗兔IgG单克隆抗体、HRP标记的山羊抗小鼠IgG单克隆抗体、小鼠抗小鼠β肌动蛋白单克隆抗体购自美国Proteintech公司,TXNRD1抑制剂金诺芬购自美国Med Chem Express公司,试剂级玉米油购自上海阿拉丁生化科技股份有限公司,小鼠CD11c阳选磁珠购自德国Miltenyi Biotec公司,小鼠脏器淋巴细胞分离液购自天津灏洋生物制品科技有限责任公司,还原型谷胱甘肽含量检测试剂盒购自北京索莱宝科技有限公司,Hoechst 33342荧光核酸染料购自美国ImmunoChemistry Technologies公司,RPMI-1640培养基、脂质过氧化传感器BODIPY™ 581/591 C11购自美国Thermo Fisher Scientific公司。
ImageQuant LAS 4000型化学发光成像分析仪、DeltaVision Ultra型高分辨率活细胞成像系统购自美国通用电气公司,CANTO PLUS型流式细胞仪购自美国Becton-Dickinson公司。
取60只C57BL/6J小鼠,参照文献[13]的手术方法行盲肠结扎穿孔(cecal ligation and puncture,CLP),分别于术后0(即刻)、6、12、24、48、72 h采用随机数字表法选取10只小鼠,脊椎脱臼处死后取小鼠脾脏,参照文献[13]的磁珠分选法,使用CD11c阳选磁珠分离纯化原代脾脏DC,常规离心计数后收集细胞进行以下观测。
裂解各时间点细胞2×106个,使用蛋白质印迹法检测细胞中TXNRD1、GPX4、SLC7A11蛋白表达。一抗分别为兔抗小鼠TXNRD1多克隆抗体(稀释比为1∶2 000)、兔抗小鼠GPX4单克隆抗体(稀释比为1∶5 000)、兔抗小鼠SLC7A11单克隆抗体(稀释比为1∶5 000)和小鼠抗小鼠β肌动蛋白单克隆抗体(稀释比为1∶1 000),二抗为HRP标记的山羊抗兔IgG单克隆抗体、HRP标记的山羊抗小鼠IgG单克隆抗体(稀释比均为1∶5 000)。以β肌动蛋白为内参照,采用ImageJ图像分析软件(美国国立卫生研究院)计算目的蛋白与内参照蛋白的灰度值比值,以此表示蛋白表达水平。本实验样本数为3。
采用比色法进行该指标检测。收集各时间点细胞1×106个,按还原型谷胱甘肽含量检测试剂盒说明书加入200 μL试剂,液氮反复冻融3次后以8 000×g离心10 min。取上清液,加入试剂后使用酶标仪测量412 nm波长处吸光度值,使用双吡啶甲酸检测各时间点上清液中蛋白浓度,按照说明书要求,根据吸光度值和蛋白浓度计算每毫克蛋白中的还原型谷胱甘肽含量。本实验样本数为3。
收集各时间点细胞2×105个,参照文献[20]的方法加入脂质过氧化传感器工作液及Hoechst 33342培养后,应用高分辨率活细胞成像系统于126倍放大倍数下观察细胞脂质过氧化水平。未发生过氧化的脂质为红色,发生过氧化的脂质为绿色,合成后绿色荧光越强表示细胞脂质过氧化水平越高。
收集各时间点细胞3×105个,加入异硫氰酸荧光素标记的小鼠源性I-A/I-E抗体、藻红蛋白标记的小鼠源性CD80抗体、藻红蛋白/花青素7标记的小鼠源性CD86抗体(稀释比均为1∶100),轻轻振荡混匀,室温避光孵育30 min后加入10 g/L多聚甲醛固定。采用流式细胞术检测DC表面标志物及共刺激分子I-A/I-E、CD80、CD86水平(以平均荧光强度表示),使用FlowJo软件(美国斯坦福大学)分析平均荧光强度。本实验样本数为3。
另取100只C57BL/6J小鼠,按随机数字表法将其分为玉米油+假伤组、玉米油+CLP组、抑制剂+假伤组、抑制剂+CLP组,每组25只。对抑制剂+假伤组和抑制剂+CLP组小鼠,参照文献[21]的给药方法和剂量,按5 mg/kg腹腔注射用玉米油稀释至2.08 mg/mL的金诺芬;对玉米油+假伤组和玉米油+CLP组小鼠行与金诺芬相同体积的玉米油腹腔注射。1 h后参照文献[13]对2个CLP组小鼠行CLP术构建脓毒症模型,对2个假伤组小鼠行假手术。采用随机数字表法从4组小鼠中各取20只单笼饲养,每24小时观察并计数小鼠存活情况,持续观察至术后7 d。采用GraphPad Prism 9.0软件绘制小鼠生存曲线图。术后24 h,取各组剩余的5只小鼠同1.2.1提取脾脏DC,同1.2.2~1.2.5进行相应检测(样本数一致)。
采用SPSS 27.0统计软件进行数据分析,计量资料数据均符合正态分布,以
小鼠细胞中TXNRD1、GPX4与SLC7A11蛋白表达于CLP术后0~24 h逐渐降低,之后逐渐升高。与CLP术后0 h比较,小鼠CLP术后24 h细胞中TXNRD1、GPX4与SLC7A11蛋白表达及CLP术后48 h细胞中TXNRD1蛋白表达均显著降低(P<0.05)。见图1与表1。
CLP术后时间点 | 样本数 | TXNRD1 | SLC7A11 | GPX4 |
---|---|---|---|---|
0 h(即刻) | 3 | 0.983±0.010 | 0.88±0.09 | 0.92±0.10 |
6 h | 3 | 0.913±0.090 | 0.79±0.09 | 0.87±0.08 |
12 h | 3 | 0.840±0.092 | 0.70±0.08 | 0.77±0.11 |
24 h | 3 | 0.615±0.117 | 0.57±0.06 | 0.55±0.09 |
48 h | 3 | 0.660±0.151 | 0.71±0.14 | 0.71±0.13 |
72 h | 3 | 0.839±0.048 | 0.77±0.12 | 0.91±0.16 |
F值 | 6.66 | 3.37 | 4.54 | |
P值 | 0.004 | 0.039 | 0.015 | |
P1值 | 0.836 | 0.643 | 0.959 | |
P2值 | 0.293 | 0.142 | 0.401 | |
P3值 | 0.002 | 0.009 | 0.008 | |
P4值 | 0.006 | 0.176 | 0.145 | |
P5值 | 0.291 | 0.484 | >0.999 | |
注:CLP为盲肠结扎穿孔,TXNRD1为硫氧还蛋白还原酶1,SLC7A11为溶质载体家族7成员11,GPX4为谷胱甘肽过氧化物酶4;F值、P值为各时间点间各指标总体比较所得;P1值、P2值、P3值、P4值、P5值分别为CLP术后6、12、24、48、72 h与CLP术后0 h各指标比较所得 |
小鼠CLP术后0、6、12、24、48、72 h细胞中还原型谷胱甘肽含量分别为(321±28)、(330±22)、(269±49)、(159±15)、(148±20)、(330±32)μg/mg,总体比较,差异有统计学意义(F=24.89,P<0.001)。与CLP术后0 h比较,小鼠CLP术后6、12、72 h细胞中还原型谷胱甘肽含量无显著变化(P值分别为0.998、0.340、0.999),CLP术后24、48 h细胞中还原型谷胱甘肽含量均显著降低(P<0.001)。
小鼠CLP术后0、6、12 h细胞脂质过氧化水平低,稍低于CLP术后72 h;CLP术后24、48 h细胞脂质过氧化水平显著高于CLP术后0、6、12、72 h。见图2。
小鼠CLP术后0~72 h,细胞中I-A/I-E、CD80和CD86水平均呈先升高后下降的趋势。与CLP术后0 h比较,小鼠CLP术后6、12、24、48、72 h细胞中I-A/I-E、CD80水平及CLP术后12、24、48 h细胞中CD86水平均显著升高(P<0.05)。见表2。
CLP术后时间点 | 样本数 | I-A/I-E | CD80 | CD86 |
---|---|---|---|---|
0 h(即刻) | 3 | 1 509±160 | 967±39 | 1 996±205 |
6 h | 3 | 4 071±155 | 3 908±96 | 1 895±64 |
12 h | 3 | 6 744±455 | 5 225±235 | 4 753±357 |
24 h | 3 | 5 580±464 | 7 369±141 | 3 563±219 |
48 h | 3 | 4 615±282 | 4 076±84 | 2 796±268 |
72 h | 3 | 2 450±70 | 1 750±100 | 1 870±251 |
F值 | 122.00 | 475.90 | 67.94 | |
P值 | <0.001 | <0.001 | <0.001 | |
P1值 | <0.001 | <0.001 | 0.979 | |
P2值 | <0.001 | <0.001 | <0.001 | |
P3值 | <0.001 | <0.001 | <0.001 | |
P4值 | <0.001 | <0.001 | 0.007 | |
P5值 | 0.011 | <0.001 | 0.949 | |
注:CLP为盲肠结扎穿孔,I-A/I-E为主要组织相容性复合体Ⅱ类亚型,CD80和CD86为白细胞分化抗原;F值、P值为各时间点间各指标总体比较所得;P1值、P2值、P3值、P4值、P5值分别为CLP术后6、12、24、48、72 h与CLP术后0 h各指标比较所得 |
术后24 h,玉米油+CLP组小鼠细胞中TXNRD1、SLC7A11与GPX4蛋白表达均显著低于玉米油+假伤组(P<0.05),抑制剂+CLP组小鼠细胞中TXNRD1、SLC7A11与GPX4蛋白表达均显著低于玉米油+CLP组、抑制剂+假伤组(P<0.05)。见图3与表3。
组别 | 样本数 | TXNRD1 | SLC7A11 | GPX4 |
---|---|---|---|---|
玉米油+假伤组 | 3 | 0.970±0.032 | 1.06±0.10 | 1.13±0.09 |
玉米油+CLP组 | 3 | 0.579±0.126 | 0.60±0.11 | 0.75±0.03 |
抑制剂+假伤组 | 3 | 0.794±0.178 | 0.79±0.04 | 1.06±0.14 |
抑制剂+CLP组 | 3 | 0.269±0.045 | 0.25±0.12 | 0.44±0.17 |
P1值 | 0.011 | 0.002 | 0.018 | |
P2值 | 0.002 | <0.001 | <0.001 | |
P3值 | 0.288 | 0.035 | 0.849 | |
P4值 | 0.038 | 0.008 | 0.045 | |
注:玉米油+假伤组、玉米油+CLP组小鼠腹腔注射玉米油1 h后分别行假手术、CLP术,抑制剂+假伤组、抑制剂+CLP组小鼠腹腔注射金诺芬1 h后分别行假手术、CLP术;TXNRD1为硫氧还蛋白还原酶1,SLC7A11为溶质载体家族7成员11,GPX4为谷胱甘肽过氧化物酶4,CLP为盲肠结扎穿孔;TXNRD1、SLC7A11、GPX4是否使用抑制剂的主效应,F值分别为14.22、32.30、8.35,P值分别为0.006、<0.001、0.020;是否进行CLP的主效应,F值分别为50.48、81.55、54.02,P值均<0.001;二者交互作用,F值分别为1.07、0.63、3.05,P值分别为0.330、0.450、0.119;P1值、P2值、P3值、P4值分别为玉米油+CLP组与玉米油+假伤组、抑制剂+CLP组与抑制剂+假伤组、抑制剂+假伤组与玉米油+假伤组、抑制剂+CLP组与玉米油+CLP组各指标比较所得 |
术后24 h,玉米油+假伤组、玉米油+CLP组、抑制剂+假伤组、抑制剂+CLP组小鼠细胞中还原型谷胱甘肽含量分别为(366±59)、(239±32)、(355±31)、(134±19)μg/mg(是否使用抑制剂的主效应,F=6.70,P=0.032;是否进行CLP的主效应,F=61.56,P<0.001;二者交互作用,F=4.52,P=0.066)。与玉米油+假伤组比较,玉米油+CLP组小鼠细胞中还原型谷胱甘肽含量显著降低(P=0.016),抑制剂+假伤组小鼠细胞中还原型谷胱甘肽含量无明显变化(P=0.987);抑制剂+CLP组小鼠细胞中还原型谷胱甘肽含量显著低于抑制剂+假伤组及玉米油+CLP组(P值分别为<0.001、0.042)。
术后24 h,玉米油+假伤组与抑制剂+假伤组小鼠细胞脂质过氧化水平低,稍低于玉米油+CLP组;抑制剂+CLP组小鼠细胞脂质过氧化水平显著高于其余3组。见图4。
术后24 h,玉米油+CLP组小鼠细胞中I-A/I-E、CD80、CD86水平均显著高于玉米油+假伤组(P<0.05),抑制剂+CLP组小鼠细胞中I-A/I-E、CD80水平均显著高于抑制剂+假伤组(P<0.05)但均显著低于玉米油+CLP组(P<0.05),抑制剂+假伤组小鼠细胞中CD86水平显著高于玉米油+假伤组(P<0.05)。见表4。
组别 | 样本数 | I-A/I-E | CD80 | CD86 |
---|---|---|---|---|
玉米油+假伤组 | 3 | 3 649±270 | 6 241±385 | 9 283±3 698 |
玉米油+CLP组 | 3 | 11 927±249 | 20 103±677 | 50 258±2 597 |
抑制剂+假伤组 | 3 | 3 463±173 | 7 287±404 | 49 051±3 438 |
抑制剂+CLP组 | 3 | 8 561±506 | 9 810±326 | 53 972±2 485 |
P1值 | <0.001 | <0.001 | <0.001 | |
P2值 | <0.001 | <0.001 | 0.283 | |
P3值 | 0.893 | 0.096 | <0.001 | |
P4值 | <0.001 | <0.001 | 0.497 | |
注:玉米油+假伤组、玉米油+CLP组小鼠腹腔注射玉米油1 h后分别行假手术、CLP术,抑制剂+假伤组、抑制剂+CLP组小鼠腹腔注射金诺芬1 h后分别行假手术、CLP术;I-A/I-E为主要组织相容性复合体Ⅱ类亚型,CD80和CD86为白细胞分化抗原,CLP为盲肠结扎穿孔;I-A/I-E、CD80、CD86是否使用抑制剂的主效应,F值分别为89.99、293.00、152.90,P值均<0.001;是否进行CLP的主效应,F值分别为1 276.00、920.00、170.20,P值均<0.001;二者交互作用,F值分别为72.10、440.60、102.90,P值均<0.001;P1值、P2值、P3值、P4值分别为玉米油+CLP组与玉米油+假伤组、抑制剂+CLP组与抑制剂+假伤组、抑制剂+假伤组与玉米油+假伤组、抑制剂+CLP组与玉米油+CLP组各指标比较所得 |
术后7 d内,4组小鼠存活率总体比较,差异有统计学意义(χ2=53.29,P<0.001)。玉米油+CLP组小鼠存活率显著低于玉米油+假伤组(χ2=17.06,P<0.001),抑制剂+CLP组小鼠存活率显著低于抑制剂+假伤组和玉米油+CLP组(χ2值分别为31.19、3.91,P值分别为<0.001、0.048)。见图5。
脓毒症作为危及生命的多器官损伤综合征,其诊治仍是临床实践面临的突出难题。DC因其抗原提呈及免疫调控能力,在脓毒症免疫过程中发挥关键作用,但也易受氧化应激及多种刺激因素影响[22, 23, 24, 25]。研究显示,脓毒症小鼠脾脏DC中活性氧含量增加,并伴随铁超载,这与多种细胞死亡形式相关[26, 27, 28]。TRX系统与谷胱甘肽系统作为机体抗氧化主要防线,其功能依赖于TXNRD1蛋白[29, 30, 31]。有资料表明,TXNRD1参与铁死亡调节,抑制其功能可显著增加细胞对铁死亡诱导剂的易感性[32, 33, 34, 35]。鉴于TXNRD1在免疫调控及对抗细胞死亡中发挥潜在作用,本研究率先探讨TXNRD1在脓毒症状态下DC铁死亡及功能改变中的可能作用及免疫学效应,旨在为精准干预脓毒症免疫失调提供新思路。
本研究采用CLP术构建小鼠脓毒症模型,结果显示术后24 h小鼠脾脏DC中抗铁死亡蛋白SLC7A11、GPX4表达及还原型谷胱甘肽水平显著降低,伴随脂质过氧化现象,提示发生铁死亡。DC大致可分为常规DC、浆细胞样DC和滤泡DC,脾脏DC主要以常规DC为主要分群,高表达的主要组织相容性复合体Ⅱ类亚型I-A/I-E等表面分子可迅速将细胞外抗原呈递给辅助性CD4+T细胞,这使得其成为高效的抗原呈递细胞[36, 37, 38]。既往研究表明,在脓毒症动物模型中,脓毒症早期DC中CD40、CD80、CD86和I-A/I-E等功能标志物显著上调以促进T细胞活化,而晚期这些表面分子表达的下调则导致免疫抑制[39, 40]。本研究中小鼠CLP术后0~12 h脾脏DC中CD80、CD86及I-A/I-E水平均显著上升,并于术后48 h明显下降,与上述结果一致。值得注意的是,小鼠CLP术后24 h脾脏DC中TXNRD1表达显著降低,与细胞铁死亡及CD80等表面分子水平降低时间点大致吻合,这三者之间可能存在联系。因此进一步实验中将CLP术后24 h作为观察时间点,参考文献[21]的操作方法给予小鼠TXNRD1特异性抑制剂金诺芬进行体内实验,以进一步验证猜想。实验结果显示,金诺芬处理后,脓毒症小鼠脾脏DC中TXNRD1、SLC7A11、GPX4蛋白表达及还原型谷胱甘肽含量显著降低,表面分子I-A/I-E、CD80水平显著下降。以上结果证实,TXNRD1在脓毒症小鼠脾脏DC铁死亡过程中具有潜在保护效应,且对DC免疫功能起到调控作用。术后7 d内存活率分析显示,TXNRD1蛋白对CLP术后小鼠具有保护作用,抑制其功能及表达导致小鼠生存率降低。
值得说明的是,尽管本实验证实了TXNRD1蛋白在铁死亡调控中的重要性,但该蛋白在脓毒症状态下表达降低及调控铁死亡的确切信号机制尚不明确。此外,由于TXNRD1缺乏相应的蛋白激动剂,本研究未能进行蛋白层面的挽救实验,且金诺芬对CD86水平影响的原因尚不可知,下一步将采用过表达与敲减策略从不同层面对TXNRD1调控DC铁死亡的具体环节进行深入研究,以期探明TXNRD1作为脓毒症免疫抑制潜在治疗靶点的可能性。
本研究结果表明,模拟脓毒症状态下,小鼠脾脏DC中TXNRD1表达降低,铁死亡增强,免疫功能减弱;抑制DC中TXNRD1表达则加重DC铁死亡及免疫功能抑制,且与脓毒症预后不良密切相关。进一步了解TXNRD1对脓毒症状态下DC的影响及其机制,将为探索脓毒症免疫应答紊乱机制与精准干预策略提供新思路。
[1] |
GalloRL,HooperLV.Epithelial antimicrobial defence of the skin and intestine[J].Nat Rev Immunol,2012,12(7):503-516.DOI: 10.1038/nri3228.
|
[2] |
Cañedo-DorantesL,Cañedo-AyalaM.Skin acute wound healing: a comprehensive review[J].Int J Inflam,2019,2019:3706315.DOI: 10.1155/2019/3706315.
|
[3] |
SorgH,TilkornDJ,HagerS,et al.Skin wound healing: an update on the current knowledge and concepts[J].Eur Surg Res,2017,58(1/2):81-94.DOI: 10.1159/000454919.
|
[4] |
GrennanD.Diabetic foot ulcers[J].JAMA,2019,321(1):114.DOI: 10.1001/jama.2018.18323.
|
[5] |
HanG,CeilleyR.Chronic wound healing: a review of current management and treatments[J].Adv Ther,2017,34(3):599-610.DOI: 10.1007/s12325-017-0478-y.
|
[6] |
AlsterTS,TanziEL.Hypertrophic scars and keloids: etiology and management[J].Am J Clin Dermatol,2003,4(4):235-243.DOI: 10.2165/00128071-200304040-00003.
|
[7] |
KischerCW,ThiesAC,ChvapilM.Perivascular myofibroblasts and microvascular occlusion in hypertrophic scars and keloids[J].Hum Pathol,1982,13(9):819-824.DOI: 10.1016/s0046-8177(82)80078-6.
|
[8] |
BarrientosS,StojadinovicO,GolinkoMS,et al.Growth factors and cytokines in wound healing[J].Wound Repair Regen,2008,16(5):585-601.DOI: 10.1111/j.1524-475X.2008.00410.x.
|
[9] |
AmiriN,GolinAP,JaliliRB,et al.Roles of cutaneous cell-cell communication in wound healing outcome: an emphasis on keratinocyte-fibroblast crosstalk[J].Exp Dermatol,2022,31(4):475-484.DOI: 10.1111/exd.14516.
|
[10] |
HanCM,ChengB,WuP.Clinical guideline on topical growth factors for skin wounds[J/OL].Burns Trauma,2020,8:tkaa035[2022-02-15].https://pubmed.ncbi.nlm.nih.gov/33015207/.DOI: 10.1093/burnst/tkaa035.
|
[11] |
ZubairM,AhmadJ.Role of growth factors and cytokines in diabetic foot ulcer healing: a detailed review[J].Rev Endocr Metab Disord,2019,20(2):207-217.DOI: 10.1007/s11154-019-09492-1.
|
[12] |
WeiY,LiJ,HuangY,et al.The clinical effectiveness and safety of using epidermal growth factor, fibroblast growth factor and granulocyte-macrophage colony stimulating factor as therapeutics in acute skin wound healing: a systematic review and meta-analysis[J/OL].Burns Trauma,2022,10:tkac002[2022-02-15]. https://pubmed.ncbi.nlm.nih.gov/35265723/.DOI: 10.1093/burnst/tkac002.
|
[13] |
ChenK,RaoZ,DongS,et al.Roles of the fibroblast growth factor signal transduction system in tissue injury repair[J/OL].Burns Trauma,2022,10:tkac005[2022-02-15]. https://pubmed.ncbi.nlm.nih.gov/35350443/.DOI: 10.1093/burnst/tkac005.
|
[14] |
LiuY,LiuY,DengJ,et al.Fibroblast growth factor in diabetic foot ulcer: progress and therapeutic prospects[J].Front Endocrinol (Lausanne),2021,12:744868.DOI: 10.3389/fendo.2021.744868.
|
[15] |
HuiQ,JinZ,LiX,et al.FGF family: from drug development to clinical application[J].Int J Mol Sci,2018,19(7):1875.DOI: 10.3390/ijms19071875.
|
[16] |
KrookMA,ReeserJW,ErnstG,et al.Fibroblast growth factor receptors in cancer: genetic alterations, diagnostics, therapeutic targets and mechanisms of resistance[J].Br J Cancer,2021,124(5):880-892.DOI: 10.1038/s41416-020-01157-0.
|
[17] |
TracyLE,MinasianRA,CatersonEJ.Extracellular matrix and dermal fibroblast function in the healing wound[J].Adv Wound Care (New Rochelle),2016,5(3):119-136.DOI: 10.1089/wound.2014.0561.
|
[18] |
desJardins-ParkHE,FosterDS,LongakerMT.Fibroblasts and wound healing: an update[J].Regen Med,2018,13(5):491-495.DOI: 10.2217/rme-2018-0073.
|
[19] |
DriskellRR,LichtenbergerBM,HosteE,et al.Distinct fibroblast lineages determine dermal architecture in skin development and repair[J].Nature,2013,504(7479):277-281.DOI: 10.1038/nature12783.
|
[20] |
TabibT,MorseC,WangT,et al.SFRP2/DPP4 and FMO1/LSP1 define major fibroblast populations in human skin[J].J Invest Dermatol,2018,138(4):802-810.DOI: 10.1016/j.jid.2017.09.045.
|
[21] |
ZhangZ,ShaoM,HeplerC,et al.Dermal adipose tissue has high plasticity and undergoes reversible dedifferentiation in mice[J].J Clin Invest,2019,129(12):5327-5342.DOI: 10.1172/JCI130239.
|
[22] |
HaenselD,JinS,SunP,et al.Defining epidermal basal cell states during skin homeostasis and wound healing using single-cell transcriptomics[J].Cell Rep,2020,30(11):3932-3947.e6.DOI: 10.1016/j.celrep.2020.02.091.
|
[23] |
Guerrero-JuarezCF,DedhiaPH,JinS,et al.Single-cell analysis reveals fibroblast heterogeneity and myeloid-derived adipocyte progenitors in murine skin wounds[J].Nat Commun,2019,10(1):650.DOI: 10.1038/s41467-018-08247-x.
|
[24] |
ZhangLJ,ChenSX,Guerrero-JuarezCF,et al.Age-related loss of innate immune antimicrobial function of dermal fat is mediated by transforming growth factor beta[J].Immunity,2019,50(1):121-136.e5.DOI: 10.1016/j.immuni.2018.11.003.
|
[25] |
ZhangX,LanY,XuJ,et al.CellMarker: a manually curated resource of cell markers in human and mouse[J].Nucleic Acids Res,2019,47(D1):D721-D728.DOI: 10.1093/nar/gky900.
|
[26] |
JinS,Guerrero-JuarezCF,ZhangL,et al.Inference and analysis of cell-cell communication using CellChat[J].Nat Commun,2021,12(1):1088.DOI: 10.1038/s41467-021-21246-9.
|
[27] |
MerrickD,SakersA,IrgebayZ,et al.Identification of a mesenchymal progenitor cell hierarchy in adipose tissue[J].Science,2019,364(6438):eaav2501.DOI: 10.1126/science.aav2501.
|
[28] |
Berry-KilgourC,CabralJ,WiseL.Advancements in the delivery of growth factors and cytokines for the treatment of cutaneous wound indications[J].Adv Wound Care (New Rochelle),2021,10(11):596-622.DOI: 10.1089/wound.2020.1183.
|
[29] |
YamakawaS,HayashidaK.Advances in surgical applications of growth factors for wound healing[J/OL].Burns Trauma,2019,7:10[2022-02-15].https://pubmed.ncbi.nlm.nih.gov/30993143/.DOI: 10.1186/s41038-019-0148-1.
|
[30] |
MaddalunoL,UrwylerC,WernerS.Fibroblast growth factors: key players in regeneration and tissue repair[J].Development,2017,144(22):4047-4060.DOI: 10.1242/dev.152587.
|
[31] |
XieY,SuN,YangJ,et al.FGF/FGFR signaling in health and disease[J].Signal Transduct Target Ther,2020,5(1):181.DOI: 10.1038/s41392-020-00222-7.
|
[32] |
LindnerV,MajackRA,ReidyMA.Basic fibroblast growth factor stimulates endothelial regrowth and proliferation in denuded arteries[J].J Clin Invest,1990,85(6):2004-2008.DOI: 10.1172/JCI114665.
|
[33] |
TaiAL,ShamJS,XieD,et al.Co-overexpression of fibroblast growth factor 3 and epidermal growth factor receptor is correlated with the development of nonsmall cell lung carcinoma[J].Cancer,2006,106(1):146-155.DOI: 10.1002/cncr.21581.
|
[34] |
HuL,ShamJS,XieD,et al.Up-regulation of fibroblast growth factor 3 is associated with tumor metastasis and recurrence in human hepatocellular carcinoma[J].Cancer Lett,2007,252(1):36-42.DOI: 10.1016/j.canlet.2006.12.003.
|
[35] |
YenTT,ThaoDT,ThuocTL.An overview on keratinocyte growth factor: from the molecular properties to clinical applications[J].Protein Pept Lett,2014,21(3):306-317.DOI: 10.2174/09298665113206660115.
|
[36] |
WernerS,KriegT,SmolaH.Keratinocyte-fibroblast interactions in wound healing[J].J Invest Dermatol,2007,127(5):998-1008.DOI: 10.1038/sj.jid.5700786.
|
[37] |
ZinkleA,MohammadiM.Structural bology of the FGF7 subfamily[J].Front Genet,2019,10:102.DOI: 10.3389/fgene.2019.00102.
|
CLP术后时间点 | 样本数 | TXNRD1 | SLC7A11 | GPX4 |
---|---|---|---|---|
0 h(即刻) | 3 | 0.983±0.010 | 0.88±0.09 | 0.92±0.10 |
6 h | 3 | 0.913±0.090 | 0.79±0.09 | 0.87±0.08 |
12 h | 3 | 0.840±0.092 | 0.70±0.08 | 0.77±0.11 |
24 h | 3 | 0.615±0.117 | 0.57±0.06 | 0.55±0.09 |
48 h | 3 | 0.660±0.151 | 0.71±0.14 | 0.71±0.13 |
72 h | 3 | 0.839±0.048 | 0.77±0.12 | 0.91±0.16 |
F值 | 6.66 | 3.37 | 4.54 | |
P值 | 0.004 | 0.039 | 0.015 | |
P1值 | 0.836 | 0.643 | 0.959 | |
P2值 | 0.293 | 0.142 | 0.401 | |
P3值 | 0.002 | 0.009 | 0.008 | |
P4值 | 0.006 | 0.176 | 0.145 | |
P5值 | 0.291 | 0.484 | >0.999 | |
注:CLP为盲肠结扎穿孔,TXNRD1为硫氧还蛋白还原酶1,SLC7A11为溶质载体家族7成员11,GPX4为谷胱甘肽过氧化物酶4;F值、P值为各时间点间各指标总体比较所得;P1值、P2值、P3值、P4值、P5值分别为CLP术后6、12、24、48、72 h与CLP术后0 h各指标比较所得 |
CLP术后时间点 | 样本数 | I-A/I-E | CD80 | CD86 |
---|---|---|---|---|
0 h(即刻) | 3 | 1 509±160 | 967±39 | 1 996±205 |
6 h | 3 | 4 071±155 | 3 908±96 | 1 895±64 |
12 h | 3 | 6 744±455 | 5 225±235 | 4 753±357 |
24 h | 3 | 5 580±464 | 7 369±141 | 3 563±219 |
48 h | 3 | 4 615±282 | 4 076±84 | 2 796±268 |
72 h | 3 | 2 450±70 | 1 750±100 | 1 870±251 |
F值 | 122.00 | 475.90 | 67.94 | |
P值 | <0.001 | <0.001 | <0.001 | |
P1值 | <0.001 | <0.001 | 0.979 | |
P2值 | <0.001 | <0.001 | <0.001 | |
P3值 | <0.001 | <0.001 | <0.001 | |
P4值 | <0.001 | <0.001 | 0.007 | |
P5值 | 0.011 | <0.001 | 0.949 | |
注:CLP为盲肠结扎穿孔,I-A/I-E为主要组织相容性复合体Ⅱ类亚型,CD80和CD86为白细胞分化抗原;F值、P值为各时间点间各指标总体比较所得;P1值、P2值、P3值、P4值、P5值分别为CLP术后6、12、24、48、72 h与CLP术后0 h各指标比较所得 |
组别 | 样本数 | TXNRD1 | SLC7A11 | GPX4 |
---|---|---|---|---|
玉米油+假伤组 | 3 | 0.970±0.032 | 1.06±0.10 | 1.13±0.09 |
玉米油+CLP组 | 3 | 0.579±0.126 | 0.60±0.11 | 0.75±0.03 |
抑制剂+假伤组 | 3 | 0.794±0.178 | 0.79±0.04 | 1.06±0.14 |
抑制剂+CLP组 | 3 | 0.269±0.045 | 0.25±0.12 | 0.44±0.17 |
P1值 | 0.011 | 0.002 | 0.018 | |
P2值 | 0.002 | <0.001 | <0.001 | |
P3值 | 0.288 | 0.035 | 0.849 | |
P4值 | 0.038 | 0.008 | 0.045 | |
注:玉米油+假伤组、玉米油+CLP组小鼠腹腔注射玉米油1 h后分别行假手术、CLP术,抑制剂+假伤组、抑制剂+CLP组小鼠腹腔注射金诺芬1 h后分别行假手术、CLP术;TXNRD1为硫氧还蛋白还原酶1,SLC7A11为溶质载体家族7成员11,GPX4为谷胱甘肽过氧化物酶4,CLP为盲肠结扎穿孔;TXNRD1、SLC7A11、GPX4是否使用抑制剂的主效应,F值分别为14.22、32.30、8.35,P值分别为0.006、<0.001、0.020;是否进行CLP的主效应,F值分别为50.48、81.55、54.02,P值均<0.001;二者交互作用,F值分别为1.07、0.63、3.05,P值分别为0.330、0.450、0.119;P1值、P2值、P3值、P4值分别为玉米油+CLP组与玉米油+假伤组、抑制剂+CLP组与抑制剂+假伤组、抑制剂+假伤组与玉米油+假伤组、抑制剂+CLP组与玉米油+CLP组各指标比较所得 |
组别 | 样本数 | I-A/I-E | CD80 | CD86 |
---|---|---|---|---|
玉米油+假伤组 | 3 | 3 649±270 | 6 241±385 | 9 283±3 698 |
玉米油+CLP组 | 3 | 11 927±249 | 20 103±677 | 50 258±2 597 |
抑制剂+假伤组 | 3 | 3 463±173 | 7 287±404 | 49 051±3 438 |
抑制剂+CLP组 | 3 | 8 561±506 | 9 810±326 | 53 972±2 485 |
P1值 | <0.001 | <0.001 | <0.001 | |
P2值 | <0.001 | <0.001 | 0.283 | |
P3值 | 0.893 | 0.096 | <0.001 | |
P4值 | <0.001 | <0.001 | 0.497 | |
注:玉米油+假伤组、玉米油+CLP组小鼠腹腔注射玉米油1 h后分别行假手术、CLP术,抑制剂+假伤组、抑制剂+CLP组小鼠腹腔注射金诺芬1 h后分别行假手术、CLP术;I-A/I-E为主要组织相容性复合体Ⅱ类亚型,CD80和CD86为白细胞分化抗原,CLP为盲肠结扎穿孔;I-A/I-E、CD80、CD86是否使用抑制剂的主效应,F值分别为89.99、293.00、152.90,P值均<0.001;是否进行CLP的主效应,F值分别为1 276.00、920.00、170.20,P值均<0.001;二者交互作用,F值分别为72.10、440.60、102.90,P值均<0.001;P1值、P2值、P3值、P4值分别为玉米油+CLP组与玉米油+假伤组、抑制剂+CLP组与抑制剂+假伤组、抑制剂+假伤组与玉米油+假伤组、抑制剂+CLP组与玉米油+CLP组各指标比较所得 |
CLP术后时间点 | 样本数 | TXNRD1 | SLC7A11 | GPX4 |
---|---|---|---|---|
0 h(即刻) | 3 | 0.983±0.010 | 0.88±0.09 | 0.92±0.10 |
6 h | 3 | 0.913±0.090 | 0.79±0.09 | 0.87±0.08 |
12 h | 3 | 0.840±0.092 | 0.70±0.08 | 0.77±0.11 |
24 h | 3 | 0.615±0.117 | 0.57±0.06 | 0.55±0.09 |
48 h | 3 | 0.660±0.151 | 0.71±0.14 | 0.71±0.13 |
72 h | 3 | 0.839±0.048 | 0.77±0.12 | 0.91±0.16 |
F值 | 6.66 | 3.37 | 4.54 | |
P值 | 0.004 | 0.039 | 0.015 | |
P1值 | 0.836 | 0.643 | 0.959 | |
P2值 | 0.293 | 0.142 | 0.401 | |
P3值 | 0.002 | 0.009 | 0.008 | |
P4值 | 0.006 | 0.176 | 0.145 | |
P5值 | 0.291 | 0.484 | >0.999 | |
注:CLP为盲肠结扎穿孔,TXNRD1为硫氧还蛋白还原酶1,SLC7A11为溶质载体家族7成员11,GPX4为谷胱甘肽过氧化物酶4;F值、P值为各时间点间各指标总体比较所得;P1值、P2值、P3值、P4值、P5值分别为CLP术后6、12、24、48、72 h与CLP术后0 h各指标比较所得 |
CLP术后时间点 | 样本数 | I-A/I-E | CD80 | CD86 |
---|---|---|---|---|
0 h(即刻) | 3 | 1 509±160 | 967±39 | 1 996±205 |
6 h | 3 | 4 071±155 | 3 908±96 | 1 895±64 |
12 h | 3 | 6 744±455 | 5 225±235 | 4 753±357 |
24 h | 3 | 5 580±464 | 7 369±141 | 3 563±219 |
48 h | 3 | 4 615±282 | 4 076±84 | 2 796±268 |
72 h | 3 | 2 450±70 | 1 750±100 | 1 870±251 |
F值 | 122.00 | 475.90 | 67.94 | |
P值 | <0.001 | <0.001 | <0.001 | |
P1值 | <0.001 | <0.001 | 0.979 | |
P2值 | <0.001 | <0.001 | <0.001 | |
P3值 | <0.001 | <0.001 | <0.001 | |
P4值 | <0.001 | <0.001 | 0.007 | |
P5值 | 0.011 | <0.001 | 0.949 | |
注:CLP为盲肠结扎穿孔,I-A/I-E为主要组织相容性复合体Ⅱ类亚型,CD80和CD86为白细胞分化抗原;F值、P值为各时间点间各指标总体比较所得;P1值、P2值、P3值、P4值、P5值分别为CLP术后6、12、24、48、72 h与CLP术后0 h各指标比较所得 |
组别 | 样本数 | TXNRD1 | SLC7A11 | GPX4 |
---|---|---|---|---|
玉米油+假伤组 | 3 | 0.970±0.032 | 1.06±0.10 | 1.13±0.09 |
玉米油+CLP组 | 3 | 0.579±0.126 | 0.60±0.11 | 0.75±0.03 |
抑制剂+假伤组 | 3 | 0.794±0.178 | 0.79±0.04 | 1.06±0.14 |
抑制剂+CLP组 | 3 | 0.269±0.045 | 0.25±0.12 | 0.44±0.17 |
P1值 | 0.011 | 0.002 | 0.018 | |
P2值 | 0.002 | <0.001 | <0.001 | |
P3值 | 0.288 | 0.035 | 0.849 | |
P4值 | 0.038 | 0.008 | 0.045 | |
注:玉米油+假伤组、玉米油+CLP组小鼠腹腔注射玉米油1 h后分别行假手术、CLP术,抑制剂+假伤组、抑制剂+CLP组小鼠腹腔注射金诺芬1 h后分别行假手术、CLP术;TXNRD1为硫氧还蛋白还原酶1,SLC7A11为溶质载体家族7成员11,GPX4为谷胱甘肽过氧化物酶4,CLP为盲肠结扎穿孔;TXNRD1、SLC7A11、GPX4是否使用抑制剂的主效应,F值分别为14.22、32.30、8.35,P值分别为0.006、<0.001、0.020;是否进行CLP的主效应,F值分别为50.48、81.55、54.02,P值均<0.001;二者交互作用,F值分别为1.07、0.63、3.05,P值分别为0.330、0.450、0.119;P1值、P2值、P3值、P4值分别为玉米油+CLP组与玉米油+假伤组、抑制剂+CLP组与抑制剂+假伤组、抑制剂+假伤组与玉米油+假伤组、抑制剂+CLP组与玉米油+CLP组各指标比较所得 |
组别 | 样本数 | I-A/I-E | CD80 | CD86 |
---|---|---|---|---|
玉米油+假伤组 | 3 | 3 649±270 | 6 241±385 | 9 283±3 698 |
玉米油+CLP组 | 3 | 11 927±249 | 20 103±677 | 50 258±2 597 |
抑制剂+假伤组 | 3 | 3 463±173 | 7 287±404 | 49 051±3 438 |
抑制剂+CLP组 | 3 | 8 561±506 | 9 810±326 | 53 972±2 485 |
P1值 | <0.001 | <0.001 | <0.001 | |
P2值 | <0.001 | <0.001 | 0.283 | |
P3值 | 0.893 | 0.096 | <0.001 | |
P4值 | <0.001 | <0.001 | 0.497 | |
注:玉米油+假伤组、玉米油+CLP组小鼠腹腔注射玉米油1 h后分别行假手术、CLP术,抑制剂+假伤组、抑制剂+CLP组小鼠腹腔注射金诺芬1 h后分别行假手术、CLP术;I-A/I-E为主要组织相容性复合体Ⅱ类亚型,CD80和CD86为白细胞分化抗原,CLP为盲肠结扎穿孔;I-A/I-E、CD80、CD86是否使用抑制剂的主效应,F值分别为89.99、293.00、152.90,P值均<0.001;是否进行CLP的主效应,F值分别为1 276.00、920.00、170.20,P值均<0.001;二者交互作用,F值分别为72.10、440.60、102.90,P值均<0.001;P1值、P2值、P3值、P4值分别为玉米油+CLP组与玉米油+假伤组、抑制剂+CLP组与抑制剂+假伤组、抑制剂+假伤组与玉米油+假伤组、抑制剂+CLP组与玉米油+CLP组各指标比较所得 |